At the heart of rocketry is a scientific principle that has been established for over 300 years. A principle so popular that is has even creeped its way into our literature – every action has an equal and opposite reaction. If you stand in front of a wall and push it hard, you will move backwards. Similarly, if you stand on a skateboard and throw a heavy object away from you as hard as you can (don’t try this at home), you will roll in the opposite direction. As you push an object forward, the object pushes back on you with the same amount of force.
In a rocket, the ‘object’ being pushed is the end product of burning fuel, which shoots out of the back of the rocket as the fuel burns, forcing the rocket to move in the opposite direction i.e. providing thrust. Early rockets used a solidified mixture of fuel and oxygen to achieve thrust due to their simple design and high thrust output. However, the lack of thrust control was a significant limitation, making them less suitable for precise manoeuvres or adjustments during flight.
History of the rocket engine
In 1926, Dr. Robert H. Goddard launched the world’s first liquid-fuelled rocket. It was powered by a combination of petrol as the fuel and liquid oxygen (LOX) as the oxidizer. The two liquids were pumped into a combustion chamber, where they were ignited to produce thrust. While it did not reach the most astonishing of heights (topping out at 12.5 metres off the ground), it laid the foundation for future advancements in space exploration.
Goddard would go on to be granted 214 patents during his lifetime. Most of them relating to rocketry. His work on liquid-fuelled rockets included innovative concepts and technologies that became foundational to modern rocketry:
1. Regeneratively-cooled engine:
Goddard designed a regeneratively-cooled engine, where the fuel was used to cool the combustion chamber before being injected into the combustion process. This helped manage the extreme heat generated during rocket propulsion.
2. Thrust vectoring:
He also introduced the concept of thrust vectoring, which involves adjusting the direction of the rocket's thrust to control its flight path. This technology is still widely used in rockets today.
3. Multistage rocket:
Although Goddard's successful launch was of a single-stage rocket, he also proposed the idea of a multistage rocket to achieve higher altitudes. This concept laid the groundwork for future developments in space travel.
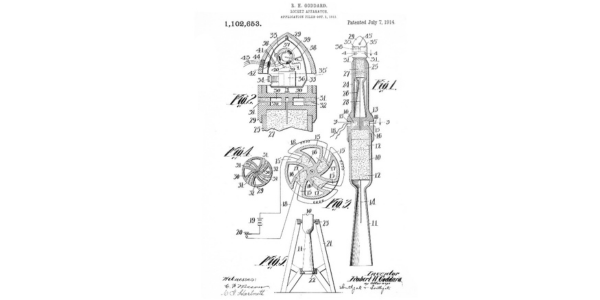
Rockets have become an icon of pop culture and even more iconic is the bell-shaped rocket nozzle that has been used in almost every rocket since the original one launched by Goddard in 1926. But this iconic nozzle was not one of Goddard’s own inventions. In fact, it was not developed for rocketry at all. The bell-shaped nozzle, or more specifically, the converging-diverging nozzle, was invented by Swedish engineer Gustaf de Laval and used for increasing the velocity of steam being supplied to the turbines of naval ships during the First World War. Nevertheless, the nozzle has been the most common choice for rocket engines due to its capabilities of efficient exhaust gas expansion, controlled transition of supersonic flow of gasses to subsonic flow and optimisation of pressure around the nozzle area to use atmospheric air for thrust.
A rocket engine typically comprises a gas turbine, a fuel pump, a combustion chamber with an opening known as the ‘throat’ and the nozzle, through which gas can escape. The pressure distribution within the chamber is asymmetric; that is, inside the combustion chamber the pressure varies little, but near the nozzle it decreases. The force due to gas pressure on the bottom of the chamber is not compensated for from the outside. The resultant force F due to the internal and external pressure difference, the thrust, is opposite to the direction of the gas jet. It pushes the chamber upwards.
To create high speed exhaust gases, the necessary high temperatures and pressures of combustion are obtained by using a very energetic fuel and by having the molecular weight of the exhaust gases as low as possible. It is also necessary to reduce the pressure of the gas as much as possible inside the nozzle by creating a large expansion ratio. The expansion ratio, is defined as the cross-sectional area at the nozzle exit divided by the area of the throat.
However, a problem arises due to the variation in ambient air pressure as the rocket ascends from sea-level into the atmosphere. It is easier to achieve a higher expansion ratio when the air is thin, but during lift-off, high expansion ratios can cause unwanted turbulences or flow separation which in turn reduces a rocket engine’s ability to generate higher thrust.
The aerospike engine
The problem was addressed first in the 1960s when Rocketdyne, a prominent rocket engine manufacturer initiated the development of an inside-out engine or an ‘aerospike’ which could provide a large expansion ratio even at sea-level. Instead of firing the exhaust out of a small hole in the middle of a bell-nozzle, an aerospike engine avoids the random distribution of exhaust gas by firing along the outside edge of a wedge-shaped protrusion, the "spike", which serves the same function as a traditional engine bell. However, unlike a bell wherein atmospheric pressure pushes exhaust gas away from the bell’s inner surface, the exhaust gas flowing along a spike is pushed towards the spike’s surface. As a result, flow separation is mitigated.
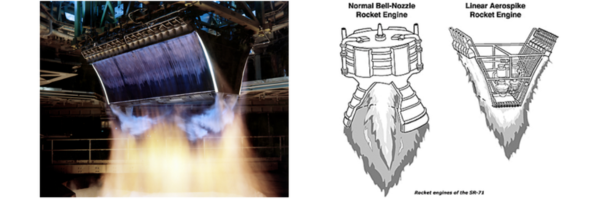
The aerospike engine has the ability to operate efficiently across a wide range of altitudes whilst providing the required level of thrust. But the biggest challenge in developing these engines lies in the complex engineering and extensive testing and validation required to make them a viable option for commercial use. Being unable to overcome the challenges it faced, the Rocketdyne project was soon abandoned due to budget constraints.
The concept was later revived by NASA in the late 1990s with the X-33/VentureStar project, which aimed to produce a reusable launch vehicle. This project also eventually fell through due to technical and financial difficulties.
In 2023, aerospace start-up Polaris Raumflugzeuge was awarded a contract by the German Armed Forces (Federal Office of Bundeswehr Equipment, Information Technology and In-Service Support) to study the potential of a linear aerospike (LAS) rocket engine as propulsion element in a spaceplane demonstrator. As part of the contract, an LAS rocket engine would be ignited and tested in flight for the first time.
The most promising application for aerospike engines in the aerospace industry are for launching payloads into orbit. The engine’s high efficiency could make space travel more cost-effective by reducing the amount of fuel needed for each launch. This could be particularly beneficial for satellite launches, interplanetary missions, and even potential manned space exploration missions.
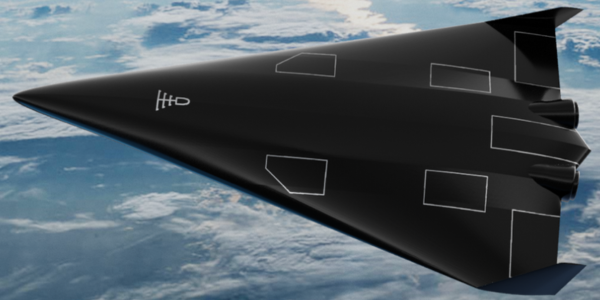
Role of additive manufacturing
A principal technological challenge in LAS rocket engines has been the engine cooling system, which can be significantly more challenging to manufacture compared to those of conventional rocket engines. However, recent advances in 3D-printing may enable new cooling concepts that were previously not feasible.
Advanced techniques in 3D printing have enabled the integration of regenerative cooling channels directly into manufactured parts. Several emerging companies such as Pangea Aerospace, are actively utilizing metal 3D printing to develop and test aerospike engine prototypes. Yet, the full potential of additive manufacturing (AM) and the flexibility in design that this technique offers is limited by the conventional means for gathering and processing data.
Hyperganic, a German company focussed on applying an AI-centred ideology to additive manufacturing, aims to bridge this gap with their Algorithmic Engineering approach. In 2022, they built the world's largest 3D-printed Aerospike rocket engine standing at 80cm tall and printed from copper on a custom-made 3D-printer.
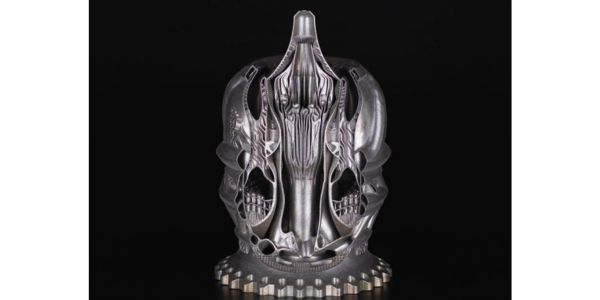
Algorithmic Engineering employs computer programming to algorithmically and autonomously generate physical objects. These algorithms serve as detailed instructions for computers to replicate the problem-solving process typically undertaken by engineers. The advantage lies in the scalability of computers, which can tirelessly refine solutions to a degree of precision beyond human capability. The objective is to accelerate innovation in physical product development, mirroring the transformative impact of Moore's Law on the computer industry.
By leveraging Algorithmic Engineering intricate designs the complement AM methodologies can be achieved. Ideally, these algorithms are informed by the limitations of the 3D printer, ensuring that generated parts can always be manufactured on that specific machine.
This approach facilitated the creation of regenerative cooling circuits, utilising dual-propellant, automatically routed across the walls of individual combustion chambers. The injector head comprises customized co-axial swirl injectors tailored to optimize performance based on their proximity to the chamber wall and the desired combustion temperature distribution.
In April 2024, the US Air Force Research Laboratory (AFRL) designed, manufactured and hot fired its first-ever, single-block 3D-printed rocket-engine thrust chamber. The chamber was manufactured using laser powder-directed energy disposition (DED) technology. The approach injects metal powder as a base material and uses a high-powered laser to construct the part in a temperature-controlled environment.
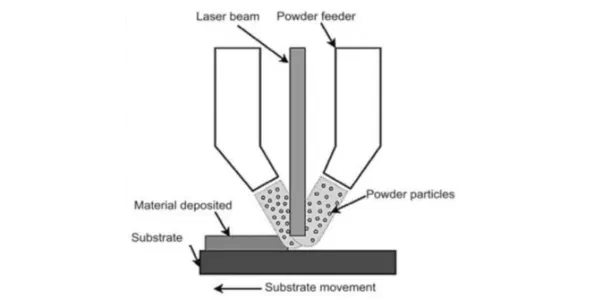
The aerospace sector is rapidly embracing additive manufacturing solutions to address design constraints. The concept involves integrating advanced AM processes into sophisticated digital engineering frameworks. This evolving landscape not only underscores the maturation of 3D-printing technologies but also highlights their growing significance in rocket production. Various methods have been explored in the past to realise the Aerospike engine, yet none have demonstrated as much potential as additive manufacturing. As the industry continues to innovate, additive manufacturing is poised to play a pivotal role in shaping the future of aerospace engineering.
Conclusion
The evolution of rocket science, epitomised by the development of aerospike engines, represents a journey marked by ingenuity and innovation. From the foundational principles laid down by pioneers like Goddard to the modern advancements in additive manufacturing, each step has propelled us closer to unlocking the full potential of space exploration.
The introduction of aerospike engines addresses longstanding challenges in rocket propulsion, offering efficient thrust across varying altitudes while promising cost-effective solutions for launching payloads into orbit. While early endeavours faced setbacks, recent initiatives signify renewed interest and potential breakthroughs in technology.
As we approach a new phase in space exploration, driven by the collective efforts of scientists and engineers, the aerospike engine represents an achievement that provides a glimpse into the future of propulsion technology.